1. Introduction
The interaction between groundwater and surface water is a significant component of the function of riparian ecosystems and sustainable water resources management (Kalbus et al. 2006). During wet periods, surface water can recharge groundwater, and during dry periods, groundwater provides a major source of water to the surface water flow. Therefore, groundwater and surface water are closely linked components of the hydrologic system. The development and utilization of any one component can affect the other, hence it is essential to understand and quantify the exchange processes between these two components for developing sustainable water resources management plans (Sophocleous 2002; Su et al. 2018).
Multi-stage hydraulic fracturing is an unconventional oil and gas well stimulation method of shale oil and gas production (ALL Consulting 2012). In most cases, water is used in hydraulic fracturing to ‘frack’ the geologic formations due to its efficiency, availability and low-cost. This technique facilitates extraction of oil and gas from vast shale reserves, which were previously considered inaccessible or unprofitable (Entrekin et al. 2011). In hydraulic fracturing, a fluid, either a liquid (most often water) or a gas, is pumped down with a suspended proppant (usually sand or ceramic beads) and additives (various chemicals which increase the performance of the fluid) under high pressure into the well, to cause the surrounding rock to fracture or crack (Oil and Gas Info 2019). When fracture pressures are abated, the proppant remains to keep the fractures open and allow oil and gas to be produced in the well. Figure 1 shows a schematic diagram of a hydraulically fractured well with horizontal drilling, where water is abstracted from surface water and groundwater sources for hydraulic fracturing. The volume of water used as fracturing fluid by the oil and gas industry varies significantly depending on the geological context. For example, in the Upper Peace Region of northwestern Alberta, Canada, the average water use per well was 3,671 m3 in 2013-2014 (Saha 2016). In 2016, the median water use per well in the Marcellus region (Pennsylvania and West Virginia) and the Permian Basin (Texas and New Mexico) was 27,950 m3 and 42,500 m3, respectively (Kondash et al. 2018). Meanwhile the average water consumption per well in the Vaca Muerta Play, Argentina in 2016, was 22,538 m3 (Rosa, D’Odorico 2019). Furthermore, in 2011-2017, the median water use per well in the Weiyuan play of the Sichuan Basin, China, was 36,014 m3 (Wu et al. 2019).
Fig. 1.
A schematic diagram of a hydraulically fractured well with horizontal drilling, and water collection from surface water and groundwater sources for hydraulic fracturing. Figure is not drawn to a scale.
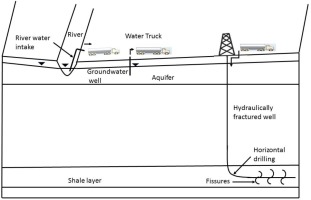
Although multi-stage hydraulic fracturing reduces the amount of natural landscape disturbance per well compared to conventional vertical drilling (ALL Consulting 2012), there is considerable public concern regarding the sustainability of this activity, because of its requirement for large volumes of water and given limited water resources (e.g. groundwater and surface water). Most often, the water is collected from surface water (i.e. rivers, lakes and wetlands), groundwater, or a combination of both. As a result, there is a need to understand the interaction between groundwater and surface water following water withdrawal for hydraulic fracturing. The objectives of this review are: a) to understand how the interaction between groundwater and surface water are affected by the impacts of water withdrawal for hydraulic fracturing, based on previously published literature, and; b) to identify future potential research in this area, required to better understand and quantify these interactions and to develop sustainable water resources management plans in shale gas and oil play regions.
2. Materials and methods
Firstly, a search for relevant previously published literature in Google Scholar was completed, using the phrase “The effects of hydraulic fracturing on” with a variety of other keywords, such as “groundwater-surface water (GW-SW) interaction, stream flow, groundwater flow, stream water level, and groundwater table”. Only publications written in the English language were considered during the review process. Secondly, the results of each publication (i.e. qualitative and quantitative) was reviewed, selecting publications that highlighted the effects of water withdrawal for hydraulic fracturing on either stream flow, groundwater table, environmental flow or surface water and groundwater availability. In total, 29 suitable publications were identified (stream flow (18), groundwater table (7), environmental flow (2), and surface water and groundwater availability (2)). In addition, publications which highlighted other environmental issues associated with hydraulic fracturing have been cited as part of this review, to demonstrate the comprehensive negative impacts of hydraulic fracturing on the environment, society and human health.
3. Results and discussion
3.1. Surface water and groundwater changes, under the effects of water withdrawal for hydraulic fracturing
Since 2010, the concerns related to hydraulic fracturing have been focused on various environmental issues, such as stray gas migration to shallow groundwater (Osborn et al. 2011; Vengosh et al. 2014) and to the atmosphere (Howarth et al. 2011), possible hydraulic connectivity between deep shale formations and shallow aquifers (Warner et al. 2012), water use (Nicot, Scanlon 2012; Carlson, Stelfox 2014), air quality (Colborn et al. 2014), seismicity (Holland 2011; Keranen et al. 2014), and the potential for contamination from hydraulic fracturing fluid and/or produced brines containing toxic substances during drilling, transport, and disposal (Dresel, Rose 2010; Rowan et al. 2011; Gregory et al. 2011). The results from these studies revealed that hydraulic fracturing has the potential for pollution of surface water, groundwater and the air, for causing earthquakes, creating conflict among competing water users and health related issues. Among these identified impacts, the potential for pollution of surface water and groundwater due to hydraulic fracturing has been paramount in public discourse and peer-reviewed journal articles (Osborn et al. 2011; Myers 2012; Barbot et al. 2013; Fontenot et al. 2013; Gordalla et al. 2013; Olmstead et al. 2013; Vengosh et al. 2013; Vidic et al. 2013; Warner et al. 2013; Brittingham et al. 2014; Vengosh et al. 2014; Gallegos, Varela 2015; Kuwayama et al. 2015). A moderate number of articles qualitatively addressed the potential impacts of hydraulic fracturing on water resources due to extensive water withdrawal from nearby water sources (Kargbo et al. 2010; Entrekin et al. 2011; Brittingham et al. 2014; Vengosh et al. 2014; Gallegos, Varela 2015; Kuwayama et al. 2015), and the combined effects of this withdrawal with other activities related to hydraulic fracturing (Rahm, Riha 2012). The potential impacts from such withdrawal include: decreased volumes of water in rivers, streams, lakes and aquifers (Rahm, Riha 2012; Brittingham et al. 2014; Vengosh et al. 2014; Gallegos, Varela 2015; Kuwayama et al. 2015); alteration of natural flow regimes (Entrekin et al. 2011; Rahm, Riha 2012; Brittingham et al. 2014); regional water shortages during periods of drought (Entrekin et al. 2011; Vengosh et al. 2014); creating conflicts among competing users in regions of water-stress (Cooley, Donnelly 2012); inadequate downstream water availability (Entrekin et al. 2011; Rahm, Riha 2012); reduced quality of water downstream for human use, due to less water availability for dilution (Entrekin et al. 2011; Brittingham et al. 2014; Vengosh et al. 2014) and; degradation of habitat (Rahm, Riha 2012; Brittingham et al. 2014) and aquatic ecosystem function (Kargbo et al. 2010; Entrekin et al. 2011; Brittingham et al. 2014), essential for wildlife. Very few studies/articles were found that quantified the impacts of water withdrawal for hydraulic fracturing, representing a significant gap in the literature.
Due to the importance of water resources, quantifying the effects of water withdrawal for hydraulic fracturing has received increased research attention over the last 7 years. The variables assessed in the selected literature included the impacts on annual stream flow (Cothren et al. 2013; Best, Lowry 2014; Sharma et al. 2015), daily stream flow (Shank, Stauffer 2015; Barth-Naftilan et al. 2015; Sullivan et al. 2015), monthly stream flow (MacQuarrie 2018; Entrekin et al. 2018), stream low flow (Sharma et al. 2015; Shrestha et al. 2016; Lin et al. 2018), environmental stream flow components (i.e. high flow, low flow and extremely low flow) (Cothren et al. 2013; Buchanan et al. 2017), annual surface water and groundwater availability (Sullivan et al. 2015; Vandecasteele et al. 2015), and annual groundwater table/level (Best, Lowry 2014; Sullivan et al. 2015; Lin et al. 2018). Studies were primarily conducted using: hydrological models, such as the SWAT (Soil and Water Assessment Tool) model (Cothren et al. 2013; Sharma et al. 2015; Shrestha et al. 2016) and the MODFLOW (Modular 3Dimensional Finite-Difference Groundwater Flow) model (Best, Lowry 2014); regression models, such as the CIRF (Conditional Inference Random Forest) model (Buchanan et al. 2017); or a range of indices such as a withdrawal index (Shank, Stauffer 2015; Barth-Naftilan et al. 2015), surface water use intensity index (Sullivan et al. 2015; MacQuarrie 2018; Entrekin et al. 2018) or water exploitation index (Vandecasteele et al. 2015).
Based on the search and review of the published literature, quantitative research most commonly focused on the impacts of water withdrawal for hydraulic fracturing on surface water. Of these, most studies considered surface water withdrawal from streams only, the key findings of which are presented in Table 1. Overall, these studies found that the total amount of water withdrawal for hydraulic fracturing was very low compared to stream flow. The magnitude of this proportion varied inversely with stream size (i.e. order) and watershed area. For example, Shank and Stauffer (2015) found daily water withdrawal for shale gas development ranged from 0.04% to 6.8% of average daily flow in the Susquehanna River Basin (71,251 km2), United States of America (USA), with the largest values associated with the headwater (i.e. upstream or lower order) streams, because of the relatively lower stream flow compared to that of higher order streams (i.e. downstream). Sullivan et al. (2015) also found similar results in the Susquehanna River Basin, USA. Barth-Naftilan et al. (2015) found daily permitted water withdrawal was less than 5% of median flow of streams that lie within comparatively large watersheds (>1000 km2) in Pennsylvania’s Marcellus Shale Play, USA. Meanwhile, MacQuarrie (2018) found monthly water withdrawal for shale gas development was 6% of mean monthly stream flow during winter months (November-February) in a sub-basin (835 km2) of the Duvernay Formation (approximately 130,000 km2) in Alberta, Canada, while for other sub-basins with larger areas, that value was lower. Furthermore, Cothren et al. (2013) found the water quantity used for hydraulic fracturing was approximately 0.05% of the overall discharge of the study area (127,300 km2) in the Fayetteville Shale Play in Arkansas, USA. Therefore, the effects of water withdrawal for hydraulic fracturing on stream flow depend not only proportionally on the amount of water withdrawn, but also inversely on the stream size and watershed area.
Table 1.
The key findings from the quantitative studies related to the impacts of water withdrawal for hydraulic fracturing on water resources.
Key finding | Justification |
---|---|
The effects of water withdrawalfrom streamsfor hydraulic fracturing on stream flow are dependent not only proportionally on the volume of water withdrawn, but alsoinversely onstream size and watershed area. | Shank and Stauffer (2015) found that daily water withdrawalranged from 0.04% to 6.8% of average daily flow in the Susquehanna River Basin (71,251 km2), USA. The largest values are associated with the headwater (i.e.upstream or lower order) streams, and vice versa. Barth-Naftilan et al. (2015) found daily permitted water withdrawalwere less than 5% of median flowof streams in the comparatively large watersheds (>1000 km2) in Pennsylvania’s Marcellus ShalePlay, USA. Similar results were found in Cothren et al. (2013), and MacQuarrie (2018). |
The effectsof water withdrawalfrom streamsfor hydraulic fracturing on stream flowaredependenton both temporal and spatial components of stream flow in the watershed. | Cothren et al. (2013) did not find any difference between the annual water bal-ance components of the baselinescenario(withnowithdrawal ofwater for hy-draulic fracturing) and the generated scenarios (with water withdrawn for hy-draulic fracturing) at basin scale (127,300 km2) in the Fayetteville Shale Play,inArkansas, USA. However, they found significant changes in stream flow during low flow months on the monthly time-step and sub-basin scale. Sharma et al. (2015) found that at the local scale (sub-basin), water withdrawalfrom streamshad little (<1.5%) impact on mean seasonal and annual flowin the Muskingum watershed (20,720 km2) of eastern Ohio, USA. However, modest (5.2% to 10.6%) impacts were found on the 7-dayminimum monthly flow (low flow) es-pecially at the local scale and in lower order streams, in comparison withbase-line and generated scenarios. Similar results were found in Best and Lowry (2014), Shrestha et al. (2016), and Entrekin et al. (2018). |
The effects of waterwithdrawalfrom streamsfor hydraulic fracturingon envi-ronmental flow of lower order streamswithsmall watershedsare significant during low flow periods. | Buchanan et al.(2017) found under the least intense withdrawal scenario (i. e. daily water withdrawal 1,210 m3)21% of reference headwaters and creeks (drainage areasless than 99 km2) experienced approximately50% reduction in stream flow during low-flow periodsin the Marcellus Shale formation (170,000 km2), USA, whereas for larger rivers,little change was found. Cothren et al. (2013) also found stream flow decreased during November 2007 and January 2008 (low flow months) by 34% and 67%, respectively, inone of the sub-basins with a large number of wells located upstream in the Fayetteville Shale Play,inArkansas, USA.Similar results were found by Shrestha et al. (2016) in the head-water streams in the sub-watersheds of the Muskingum watershed, USA. |
The effects of water withdrawalfrom groundwater for hydraulic fracturing on groundwater levelare local, and groundwa-ter leveldecreasesproportionally tothe amount of water withdrawalfrom the groundwater pumping well. | Best and Lowry (2014), Sullivan et al. (2015), Vandecasteeleet al. (2015), and Lin et al. (2018) found notable groundwater level decreases near the pumping wells. |
In addition, the effects of water withdrawal for hydraulic fracturing on stream flow depends on the temporal and spatial stream flow in the watershed. For example, Cothren et al. (2013) found no difference between the annual water balance components of the baseline scenario (with no water withdrawn for hydraulic fracturing) and the generated scenarios (with water withdrawn for hydraulic fracturing) at basin scale (127,300 km2) in the Fayetteville Shale Play in Arkansas, USA. However, on the monthly time-step and sub-basin scale, they found significant changes in stream flow during low flow months. Sharma et al. (2015) found at the local scale (i.e. sub-basin), water withdrawal from streams had little (less than 1.5%) impact on mean seasonal and annual flow in the Muskingum watershed (20,720 km2) of eastern Ohio, USA. However, modest impacts were found on the 7-day minimum monthly flow (low flow), especially at the local scale and in lower order streams, which had a variable impact between 5.2% and 10.6%, in comparison to baseline and generated scenarios. They also found the impacts of water withdrawal from streams is greater on the 7-day minimum monthly flow than on the mean monthly, seasonal and annual stream flow, because of the lower value of the 7-day minimum monthly flow. In the same watershed, Shrestha et al. (2016) found that the headwater streams in the sub-watersheds were heavily affected, with significant decrease in 7-day low flow. Best and Lowry (2014) found under the maximum density of well development, stream flow was reduced by up to 13% in a localized region with narrow valleys and streams with lower annual discharge, whereas stream flow reduction still remained under 3% throughout most of the stream network in a study area (3,390 km2), in the Marcellus Shale Play in New York, USA. Entrekin et al. (2018) found estimated daily water usage for hydraulic fracturing exceeded the monthly low-flow withdrawal threshold in 48-79% of the catchments (mainly in smaller catchments) during dry months (June-November) in 2011-2014, in the Fayetteville Shale Play (total area: 127,300 km2, consists of catchments ranged in size from 47 to 1,959 km2) in Arkansas, USA. Lin et al. (2018) found during low flow conditions, the small-to-medium sized streams in the Bakken Shale Play (35,000 km2) in North Dakota, USA, had more stream flow during 2008-2014 than 2000-2007, due to greater rainfall than normal. Therefore, the Bakken Shale Play oil development had little impact on stream flow. Using a water exploitation index, Vandecasteele et al. (2015) evaluated the potential impacts of shale gas development in northern Poland. They found that in 2028 under the high scenario (i.e. water consumption per well 19,000 m3), 0.83% of the hydraulic fracturing water would come from surface water, while 22.42% would come from groundwater sources. It was concluded that water for shale gas extraction in northern Poland should be withdrawn from surface waterbodies, in order to reduce stress on groundwater. Alternatively, in 2011, Sullivan et al. (2015) found 77.5% and 21.9% of hydraulic fracturing water was taken from surface water (i.e. rivers and stream) and groundwater, respectively, in the Susquehanna River Basin, USA. It indicated that groundwater should be used for hydraulic fracturing to reduce impact on surface water in this basin.
Water withdrawal from streams for hydraulic fracturing has significant effects on environmental flow (i.e. instream flow needs) of lower order streams with small watersheds, during periods of low flow. According to the Brisbane Declaration (2007), environmental flow is defined as “the quantity, timing, and quality of water flows required to sustain freshwater and estuarine ecosystems and the human livelihoods and well-being that depend on these ecosystems.” Buchanan et al. (2017) revealed water withdrawal for shale gas development alters natural flow regimes, especially streams draining small watersheds (less than 99 km2) during low-flow periods in the Marcellus Shale formation (170,000 km2), USA. They found under the least intense withdrawal scenario (i. e. daily water withdrawal 1,210 m3) 21% of reference headwaters and creeks (drainage areas less than 99 km2) experienced approximately 50% reduction in summer flow (low flow months), whereas for larger rivers little change was found. Cothren et al. (2013) found at one of the sub-basins with a large number of wells located upstream in the Fayetteville Shale Play in Arkansas, USA, stream flow decreased during November 2007 and January 2008 (low flow months) by 34% and 67%, respectively. Similar results were found in the headwater streams in the sub-watersheds of the Muskingum watershed, USA (Shrestha et al. 2016). Therefore, lower order streams in a smaller sub-basin (sub-watershed) are at greater risk to be impacted negatively by water withdrawal for hydraulic fracturing during intervals of low flow or water scarcity, such as during winter months or periods of drought (Vandecasteele et al. 2015; MacQuarrie 2018). Our searches revealed scant published literature on the impacts of water withdrawal for hydraulic fracturing on other types of surface water bodies, such as lakes or wetlands. Water withdrawal from such sources requires additional attention, as lakes and wetlands can ease the negative impact of flooding and droughts by storing large amounts of water which is then released during shortages. They also replenish groundwater, improve water quality of downstream watercourses, and preserve the biodiversity and habitat of the surrounding area.
Similarly, we found very little research on the impacts of water withdrawal for hydraulic fracturing on groundwater. Studies which did assess the effects on groundwater, found that groundwater level decreased locally and proportionally to the amount of water withdrawn from the groundwater pumping well (Best, Lowry 2014; Sullivan et al. 2015; Vandecasteele et al. 2015; Lin et al. 2018). For example, Lin et al. (2018) found regional groundwater level decreased by 0.3-1.5 m in three shallow aquifers, where a large number of hydraulically fractured wells used groundwater from those aquifers during fracturing operation in the Bakken Shale Play, USA. However, one study using the MODFLOW model indicated that groundwater level (for the study area of 3,390 km2) did not experience any detectable change when water was withdrawn from the stream network (Best, Lowry 2014). This finding is likely a result of the coarser model resolution which consisted of 193 rows and 281 columns of 250 m × 250 m cells. However, finer grid resolution (e.g. 10 m by 10 m grid cells) of the model domain could detect groundwater levels changes (i.e. declines) near to the surface water withdrawal locations.
Based on our literature search, very few research studies have used hydrological models to quantify the effects of water withdrawal on water resources. These studies often made a range of assumptions due to missing information (i.e. location of water withdrawal, type of water source, amount of water per well, and timing of water withdrawal), which are detailed in Table 2. They simulated annual stream flow, stream low flow, environmental steam flow components (i.e. high flow, low flow and extremely low flow) and annual groundwater table/level under the influence of water withdrawal for hydraulic fracturing. On the other hand, studies which used regression models (e.g. CIRF model) simulated only environmental stream flow components (i.e. high flow, low flow and extremely low flow). Furthermore, studies which used a withdrawal index, surface water use intensity index, or water exploitation index, estimated the proportion of the water withdrawn from stream for hydraulic fracturing relative to the stream flow in relation to the orders of streams and the overall discharge of the study area.
Table 2.
Assumptions made in hydrological models of reviewed studies due to missing information, to quantify the impacts of water withdrawal for hydraulic fracturing on water resources.
Study | Type of water source | Location of water withdrawal | Amount of water per well | Timing of water withdrawal |
---|---|---|---|---|
Cothren et al.(2013) | Stream (surface water) | From the nearest stream to each hydraulically fractured well | 18,927.0 m3 | 3,785.4 m3of water was uniformly withdrawn during 5 consecutive months |
Best,Lowry (2014) | Stream (surface water) and groundwater | For surface water with-drawal: from the nearest stream to each hydrau-lically fracturedwell; For groundwater withdrawal: from the closest munici-pal well and private well to each hydraulically fractured well | 11,356.2 m3 | All water withdrawals were distributed over the entire year |
Sharma et al.(2015) | Stream(surface water) | No information reported | The amount of water for each hydraulically frac-tured well reported in www.fracfocus.org was used for each hydrau-lically fractured well | No information reported |
Shrestha et al.(2016) | Stream(surface water) | No information reported | The amount of water for each hydraulically frac-tured well reported in www.fracfocus.org was used for eachhydrau-lically fractured well | No information reported |
3.2. Potential opportunities for future research
This review has identified missing information and gaps in the current understanding of GW-SW interaction associated with the effects of water withdrawal for hydraulic fracturing. The following research areas are considered as potential opportunities for future study:
How the mechanism and quantity of water withdrawal from surface water (i.e. streams) and groundwater sources for hydraulic fracturing might affect groundwater discharge (i.e. base flow), surface water and groundwater levels, and groundwater contributions to stream flow in shale gas and oil play areas. In line with this, questions for consideration should include: What are the temporal patterns of those impacts throughout the year and what are the spatial patterns of those impacts in a shale gas and oil play area? Since previous studies have demonstrated that water withdrawal from streams has little impact on stream flow in comparatively large watersheds, further research should be done at a small scale (e.g. streams with small catchment areas) to better understand localized GW-SW interaction and quantify the impacts of water withdrawal from surface water (streams) and groundwater for hydraulic fracturing. In addition, models should use a finer grid resolution (e.g. 10 m by 10 m grid cells) to understand and visualize those impacts on groundwater level changes, near to the surface water withdrawal locations in stream.
What are the temporal and spatial patterns of the impacts of water withdrawal for hydraulic fracturing on other surface water bodies, especially lakes and wetlands?
What are the potential impacts of water withdrawal for hydraulic fracturing on other water users, such as agriculture, mining, manufacturing industries, municipal water supply, especially in regions with limited water resources?
Do we need to improve the definition of passby flow for lower order streams, especially during periods of low flow because the relative reduction in stream flow is higher in those streams due to water withdrawal? Passby flow is defined as the minimum stream flow that must be allowed to pass through a prescribed point of stream at any time, during which a water withdrawal is occurring from that point (Liu et al. 2018). When the natural flow is below the threshold passby flow at a prescribed point of stream, water withdrawal must be stopped from that point, and the entire natural flow must be allowed to pass through that point of stream.
Can pressure changes in hydraulic fracturing activate fracture connections to change flow to or from streams/lakes/wetlands, which might affect GW-SW interaction? This research should be conducted at a small scale to understand and quantify any variation.
Addressing these questions would provide comprehensive information needed to develop regional water budgets, which will help regional water managers to develop sustainable water resources management plans in shale gas and oil play regions. For example, the temporal (i.e. monthly, seasonal, annual) changes in groundwater contributions to stream flow could determine the temporal status of groundwater resources and site conditions for groundwater-dependent terrestrial ecosystems (Naumburg et al. 2005). Determination of the monthly, seasonal and annual variations in the dependency of stream flow on groundwater, together with temporal surface water flow, would provide useful information to set monthly, seasonal and annual water extraction limits from the rivers, lakes and groundwater, and aid allocation to stakeholders for future water supply. In addition, a better understanding of GW-SW interactions will help water managers to take proper actions to maintain healthy aquatic and terrestrial ecosystems. For example, suspending water licenses for water withdrawal from local water sources during periods of low flow and drought, recycling water for hydraulic fracturing, promoting hydraulic fracturing during high flow and water level in rivers, streams and lakes, building water reservoirs, and encouraging the use of alternative fracturing fluids (e.g. frac oil; a combination of frac oil and nitrogen; a combination of frac oil and carbon dioxide, or; a combination of frac oil, acid water and hydrochloric acid) in regions with limited water resources during periods of low flow and drought.
The oil and gas industry currently uses a range of conservation practices for hydraulic fracturing to manage water resources, which mainly fall into three categories: 1) using lower quality water from non-traditional sources, 2) recycling and reusing produced and flowback waters, and 3) building infrastructure for transporting water (American Petroleum Institute 2017). Here, produced water means the water naturally present in the geologic formation, flowing to the surface along with oil and gas when those are pumped from the well (Freyman 2014), and flowback water refers to the water injected into the well as a fracturing fluid comes back to the surface after the end of hydraulic fracturing. The choice to use each practice(s) is based on a variety of factors, such as local water stresses, individual business needs, and the particular requirements for specific geologic formations. In Canada, the oil and gas industry uses deep saline and non-potable groundwater (Encana 2013), water reservoir and lined water storage pits (Chevron Canada 2019), water resource hub that recycle produced water (Encana 2013), water treatment plants (Encana 2019), recycling and reusing produced and flowback waters (BC Oil & Gas Commission 2012). In the USA, recycling and reusing of produced and flowback waters is very common (U.S. Chamber of Commerce Foundation 2019), as well as buying effluent water from local municipalities (Freyman 2014).
There are significant challenges to overcome in order to address the identified research gaps. The major challenge results from limited data with which to assess the effects of water withdrawal for hydraulic fracturing on GW-SW interaction. For example, the limited number of groundwater monitoring wells and surface water monitoring gauges is one concerning issue. This problem is more significant in Canadian shale gas and oil play regions (i.e. Alberta and British Columbia) than in the USA. For example, in the Duvernay Formation (approximately 130,000 km2) in Alberta, Canada, there are publicly available records for only 60 active groundwater monitoring wells, accessible from Alberta provincial groundwater monitoring wells database (http://environment.alberta.ca/apps/GOWN/), and 10 surface water monitoring stations, which are maintained by the Water Survey of Canada (https://wateroffice.ec.gc.ca/).
In addition, monitoring stations of surface water bodies are primarily in streams and rivers, with very few in lakes and wetland areas. Furthermore, datasets that cover a wide spatial distribution of hydraulically fractured wells are not available for longer time periods (e.g. 10 years). For example, in the USA, this information has been available in the national hydraulic fracturing chemical registry called “fracfocus.org” since April 11, 2011 (United States Environmental Protection Agency 2015). Whereas in Canada, this data is accessible from a publicly available chemical disclosure registry called “fracfocus.ca” since January 1, 2012 in British Columbia, and December 19, 2012 in Alberta (Rivard et al. 2014). Another issue arises from a lack of data on the timing of water withdrawal for each well, the type of water source for hydraulic fracturing, and the locations of the water source in many shale gas and oil play regions. Having more comprehensive and readily available data would greatly assist in addressing the identified research gaps more accurately, through use of more detailed hydrological models.
Currently, there are no specific regulations in the USA or Canada specific to water withdrawal for hydraulic fracturing activities. Richardson et al. (2013) conducted a survey of regulations in 31 states where sources of shale gas and oil are available or might be available, and found that several states had discussed rules and restrictions for water withdrawal specific to shale gas and oil industry, however only in draft form. It was found that none had passed such legislation, and so these 31 states regulate water withdrawal (both surface water and groundwater) under general regulations. Meanwhile, 13 states (Texas, Utah, Colorado, Nebraska, Wyoming, Kansas, Oklahoma, New Mexico, California, Arkansas, Maryland, Pennsylvania and North Dakota) require water permits for all water withdrawal activities. 4 states (Michigan, New York, Georgia and New Jersey) require water permits only for water withdrawal over a specific threshold at 378.54 m3 per day or more. Similarly, Montana, South Dakota, Mississippi and Kentucky require permits over 190.78 m3, 98.12 m3, 75.70 m3 and 37.85 m3 per day, respectively. Virginia has the highest threshold, requiring permits for water withdrawal of 1,135.62 m3 per day or more. More stringently, 3 states (Indiana, Ohio, and Vermont) require permit, registration and reporting over a specific threshold. While Indiana requires permit, registration and reporting of withdrawal over 378.54 m3 per day, Ohio and Vermont require registration and reporting for water withdrawal over 378.54 m3 and 75.70 m3 per day, respectively, but also require permits only when water withdrawal is more than 7,570.82 m3 and 218 m3 per day, respectively. West Virginia, Illinois, Alabama, Tennessee, and North Carolina do not require any permits, but mandate registration and reporting for water withdrawal for over 26.50 m3, 378.54 m3, 378.54 m3, 37.85 m3 and 378.54 m3 per day, respectively. Comparably, Louisiana does not provide any permits, but requires registration and reporting for all water withdrawals.
In Canada, there are also no water withdrawal regulations specific to hydraulic fracturing. For example, in Alberta and British Columbia where the majority of hydraulic fracturing activities occur, the oil and gas operators have to submit their water license applications along with their requested water demand for a specific year (e.g. in British Columbia 3 years projection period). The request for water demand will consider the numbers of wells to be completed in a specific period, how much water will be required, if there will be any recycling and which sources (i.e. groundwater and/or surface water) and locations will be used for hydraulic fracturing activities. The corresponding authorities (Alberta Energy Regulator in Alberta, and British Columbia Oil and Gas Commission in British Columbia) determine the outcome of applications depending on available water resources. Alternatively in Saskatchewan and Manitoba, water licenses are required for industrial activities when water withdrawal is over 4,933.92 m3, and 25 m3 per day, respectively.
4. Conclusions
This review discussed and evaluated the context and effects of water withdrawals for hydraulic fracturing on surface water and groundwater resources, based on previously published literature. It was found that water withdrawal for hydraulic fracturing has negative impacts on the volume of water resources, and that most of the quantitative research focused on surface water sources, particularly focusing on water withdrawal from streams only. The key findings of those quantitative studies include: 1) the effects of water withdrawal from streams for hydraulic fracturing on stream flow depend not only proportionally on the volume of water withdrawn, but also inversely on the stream size and watershed area; 2) the effects of water withdrawal from streams for hydraulic fracturing on stream flow depend on both temporal and spatial components of stream flow in the watershed; 3) during periods of low flow, water withdrawal from streams for hydraulic fracturing have significant effect on environmental flow of lower order stream in small watershed, and; 4) groundwater level decreases locally and proportionally to the volume of water withdrawn from the groundwater pumping well for hydraulic fracturing. This review also demonstrates that there is a lack of published research that quantifies changes in the GW-SW interaction under the effects of water withdrawal for hydraulic fracturing. Based on this, suggestions for further research are made, alongside identifying the current data limitations that constrain robust analysis. In particular, the effects of water withdrawal on lakes and wetlands during periods of low flow, and in areas of groundwater flow, require further investigation. Natural gas production using hydraulic fracturing is expected to increase globally to meet future energy demands. Given the extensive use of water, a much better understanding of the effects on watersheds and hydrologic function, is required. The development of sustainable water resource management plans calls for a much better understanding of GWSW interactions.